A new “smart” inverter to help accelerate the transition to renewables – developed by Tapestry and CSIRO and supported by the Digital Future Initiative
The following case study was originally published by Tapestry
The challenges facing today’s inverters
Inverters are an essential part of the world’s transition to clean energy — transforming renewable electricity into usable power. They do this by converting the direct current (DC) electricity from energy sources like wind and solar into alternating current (AC) with voltage and frequency that can be safely used to power devices. Without inverters, it would be impossible to integrate renewable resources into the electric grid.
Despite being referred to as the “brains” of devices like wind turbines, solar panels, batteries, and electric vehicles, inverters have been historically underutilized. This is because they’re expensive, less efficient than traditional generators, and have contributed to both high-profile blackouts and catastrophic fires.
The team at Tapestry, X’s moonshot for the electric grid, was curious to prototype a new kind of inverter that could be used to not only increase renewable energy usage, but also stabilize the grid. The team is developing a set of AI-powered tools to help plan, build, and operate a fully-decarbonized grid, and seeks out innovative partners around the world as they develop and deploy their technology. With the highest per-capita rooftop solar in the world, Australia was a natural setting to explore how a new advanced inverter could help us transition to a greener, more reliable future grid.
“Australia has had incredible success in the clean energy space due to both its location and its scientific research,” explains Dr. Leo Casey, Tapestry’s Chief Scientist and inverter expert. Leo brings 40 years of experience in renewables and inverters to Tapestry, including as founder of the inverter manufacturing company Satcon. More recently, he published a paper that explored the potential of advanced inverters to address many of the issues associated with legacy inverter controls.
“Advanced inverters will play a fundamental role as the world transitions to clean energy,” Leo explains. “They can help us build a big, stable grid using renewables.”
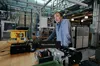
Dr. Leo Casey, Tapestry’s Chief Scientist in the moonshot factory’s labs in Mountain View California
With the support of Google Australia’s Digital Future Initiative – a $1B investment in Australian infrastructure, research, and partnerships—Tapestry began collaborating with the energy team at CSIRO, Australia’s national science agency. Together, the teams have been exploring how advanced inverters could help accelerate the country’s clean energy transition. CSIRO has a century-long history of innovation, including inventing Wi-Fi, and is also a partner to the Australian Centre for Advanced Photovoltaics, which pioneered much of the foundational research underlying today’s solar power technology.
The CSIRO team acutely understands the need for advanced inverter technology. Although Australia’s electricity mix is still dependent on fossil fuels for more than two-thirds of its power, the country’s plentiful wind and solar resources have enabled clean energy sources to grow rapidly in recent years. Renewables have even provided more than 70 percent of the country’s demand for short periods. This need to manage a complex, highly distributed, and rapidly-evolving grid has put Australia on the front lines of the energy transition.
“We’re sitting on a goldmine of renewable energy,” says Dr. Stephen Craig, CSIRO’s smart energy lead. “Yet much of it is unused due to our inability to harness it effectively.”
In order to transition to a grid that relies on renewables, the Tapestry and CSIRO teams have been working to design an advanced inverter that is faster, more responsive, and able to coordinate with devices across the grid to maintain stability in most conditions.
Managing the “delicate flow of electricity”
To understand the importance of inverters to the grid of the future, it’s helpful to first understand how today’s electric grid has functioned for more than a century. The traditional foundation of the grid has been composed of large, centralized coal or natural gas-fueled power plants that produce electricity by spinning large, magnetized iron turbines surrounded by copper wire to generate current.
These “synchronous” generators speed up or slow down in response to changes in supply or demand. For example, if people begin using more electricity around 6 p.m. after arriving home from work and turning on lights and televisions, the grid’s frequency (or speed) temporarily dips due to an increase in demand.
As a result, generators in other locations will pick up the slack, and all of the generators will spin a bit faster in order to maintain a safe tempo for the grid as a whole. Because of the considerable physical inertia of these large, heavy turbines, their output changes slowly and gradually in response to the constantly-fluctuating conditions on the grid, providing the system with built-in stability.
Wind, solar, and batteries replace the existing slow-and-steady dynamic with electricity sources that can turn on and off very quickly, much faster than a second—for instance, if a cloud passes over a solar panel. And instead of a small number of very large power plants connected to the long-distance transmission system, these clean energy resources can be located anywhere on the grid, and can range from massive wind and solar farms with hundreds of megawatts of capacity, to a small solar and battery installation on a farmhouse at the very end of a distribution line.
“These inverters delicately manage the flow of electricity from myriad small generators, ensuring a steady supply and demand balancing,” explains Dr. Julio Braslavsky, Principal Research Scientist at CSIRO. “Much like how capillaries regulate blood flow throughout the body.”
Renewable resources need inverters to convert their variable, and at times highly variable, DC output to AC electricity at the appropriate frequency and voltage.
“The contrast between synchronous generators and inverter-based renewable resources essentially represents a transition from the analog power world to the digital power world,” explains Leo. “The grid is evolving from a relatively simple configuration with built-in physical stability to an exponentially more complex, widely-distributed, and fast-changing system that will rely on sophisticated computing and algorithms to harness its transformative potential.”
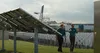
Tapestry’s Dr. Leo Casey and CSIRO Lead Scientist, Dr. Julio Braslavsky, at CSIRO’s labs in Newcastle, Australia
Moving from grid-following to grid-forming inverters
The inverters used in most clean energy resources today are relatively fragile devices made of silicon. These devices rely on simple “grid-following” algorithms, which means that they use sensors to “follow” conditions on the grid from moment to moment, determining the frequency and voltage required from the grid itself. However, these grid-following inverters operate in isolation, only able to “see” what’s in front of them. They lack the smarts to sense the status of other devices across the grid or proactively respond to changing conditions in order to maintain a steady flow of safe power.
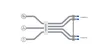
Grid-following inverters can only "see" what's in front of them and can’t communicate with each other or with other devices across the grid. This can be a problem when too much energy comes from renewable sources.
These devices generally suffice for integrating a limited number of large-scale wind and solar farms, whose output is typically determined by a site controller that manages a number of central inverters. But the rapid growth of numerous, smaller-scale, and geographically-distributed renewables on some grids—such as houses adding solar or electric vehicles with bidirectional charging capability—has revealed the limitations of the present day inverters.
While inverters can change their output much faster than synchronous generators, they aren’t able to safely manage their own rate of change of frequency (or speed) in the context of a complex, massively-distributed system populated by multiple other inverter-based resources. For example, an inverter may misinterpret a fluctuation in voltage as a grid fault, and respond by turning off as a protective measure, resulting in a dangerous chain reaction of inverters all switching off simultaneously, leading to power outages. The grid also risks becoming destabilized if inverter-based resources all inject power at the same frequency and voltage at the same time when recovering from a blackout or brownout.
Grid-following resources try to actively inject power, rather than passively allowing the loads to determine the power. And because present inverter technology is grid-following, it needs the grid to be “on,” so it can follow along and operate properly. This means that these devices cannot function independently of each other, or restart the grid during a blackout.
Today’s inverters also suffer from a materials challenge. These silicon-based devices can struggle to manage the heat from the continuous conversion of high-voltage and high-power levels, which leads to wasted energy due to poor conversion efficiency. In some cases, this can even cause fires or, in extreme situations, explosions from overheating.
For clean energy to replace fossil fuels, inverters will need to reliably keep the lights on and the grid stable, even during challenging conditions like heat waves or polar vortexes.
In order to maintain a stable and reliable power supply we need faster, smarter inverters that are “grid-forming” instead of “grid-following.” Grid-forming means they can communicate with other devices like solar panels, batteries, and traditional generators on the grid, determine the state of these other devices, and work together to keep the grid stable. They can even blackstart the larger grid in the event of a system-wide blackout.
“Unlike their less savvy predecessors, grid-forming inverters can communicate with the grid,” explains Dr. John Ward, Energy Systems Research Director at CSIRO. “They can discern precisely when to ramp up energy distribution.”
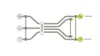
Grid-Forming Inverters can communicate with other devices like solar panels, and batteries, determine the state of these other devices, and work together to keep the grid stable.
While there are many efforts underway to develop cost-effective smart inverters, to date, the technology essentially remains in the prototyping and demonstration phase. Researchers have struggled to find a path to commercialization against today’s cheap, mass-produced inverters.
A pathway to smart, affordable inverters
As the Tapestry team looked to bring more “smarts” to inverters, they drew on X the moonshot factory’s experience embedding intelligence into physical systems, such as creating cars that drive themselves with Waymo, or autonomous drones that deliver packages with Wing.
Leo saw an opportunity to compensate for the inherently more fragile and less rugged nature of electronic inverters, with embedded intelligence. “The inverter has more brains than your computer, and we don’t do anything with it outside of converting energy and putting it on the grid,” he says. “We can create smart inverters that are aware of how wind moves and light changes, and are responsive to dynamic conditions. They can also compensate for instances in which they might normally fail, and react in a protective way instead.”
Beyond replacing synchronous generators, pairing this intelligence with highly granular, dynamic models of the entire grid—a broader goal of the Tapestry team—could enable massively distributed clean energy resources to operate in concert, delivering a more cost-effective, reliable performance.
After spending several years experimenting with ways to create a smaller, cheaper, more reliable, and more powerful inverter, Leo was inspired by technological breakthroughs in an unexpected place: the automotive industry.
“The foundation of this advanced inverter was originally designed for electric cars,” he explains. “The motor drives used in electric vehicles are similar to inverters used on the grid, but there are some very important differences which we have designed around.”
Leo and the Tapestry team believe that by leveraging the cost efficiencies of the automotive supply chain, the motor drives have the potential to serve as a platform for dramatically less expensive advanced inverters. Tapestry’s prototype could also reduce costs because it uses silicon carbide instead of the silicon used in conventional inverters. Despite silicon carbide’s higher cost per weight than silicon, its ability to withstand dramatically elevated operating temperatures and voltages than silicon-based devices could lead to significant overall system savings.
The advanced inverter design also benefits from the motor drive’s built-in intelligence, which allows it to more accurately detect grid conditions. Crucially, it also allows these inverters to be programmed remotely, enabling them to be continually modified for improved performance and new functionality in the same way that electric vehicles can receive over-the-air upgrades.
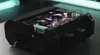
A prototype of Tapestry’s advanced inverter in the CSIRO lab. It’s roughly the size of a laptop computer.
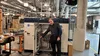
Dr. Leo Casey standing in front of a traditional inverter in Tapestry’s labs in Mountain View.
Promising early results
With the support of Google Australia, CSIRO researchers have been prototyping the advanced inverter designed by Leo at its Newcastle Energy Centre, which focuses national research on future low emission technologies, including sustainable fuels, energy storage and energy system integration. The novel design features signal sensing and signal filtering hardware, as well as grid forming and microgrid software so that the device can not only more accurately detect voltages and currents, but also act on that information.
After running a number of tests in the lab, the teams are encouraged by the early results. The advanced inverters demonstrated they could coordinate with devices across the grid to maintain stability, they could be 50 percent more cost-effective to produce, and they don’t compromise on conversion efficiency.
In a global first, the teams also demonstrated that their prototype can seamlessly transfer models and simulations to physical inverters. “The ability to accurately model and simulate grid behavior is an increasingly important element of the decarbonization effort, and something that Tapestry has worked on for years,” Leo says. “It is essential for our overall mission to virtualize the power system with precision and accuracy, so that it’s much easier for everyone who works with the grid to plan and manage.”
In the next phase of their tests, Tapestry will also be exploring opportunities to deploy these advanced inverters in the field with trusted power distribution partners to test how well they function in the real world. “These tests and the next phases of our work together are promising,” Leo says. “They will enable us to transition more quickly to a zero-emission grid that is not only cleaner, but more resilient and equitable as well.”
Google Australia’s Scott Riddle adds that the collaboration exemplifies the best of what his organization has set out to do. “Google’s Digital Future Initiative was established to catalyze impactful partnerships like this,” he says. “We’re excited by the potential Tapestry and CSIRO’s work has to accelerate the transition to renewables not just here in Australia, but around the world.”
“This work with Tapestry builds on CSIRO’s 20+ years of research on Australia’s energy system, emissions reductions and economic futures,” says CSIRO Energy Director Dr. Dietmar Tourbier. “The transitions required for Australia to meet its emissions commitments and remain globally competitive are complex and solutions require collaboration across industry, government, finance, and the global energy sector.”
In the meantime, for industry experts like Leo, Australia serves as an optimal testing ground. “For me, it has always been about renewables,” he says. “Australia is an ideal place to work on grid-connected renewables, so we are fortunate for our collaboration with CSIRO. I’m thrilled to see where this will go next.”